Passives Series - Part 1 - Ferrites
One of the most effective methods of suppressing electronic noise is with the use of ferrite cores. Ferrite cores are used in a wide range of applications, including microwave devices and RF applications, as well as electronic noise reduction in cables. This module introduces the concepts of ferrites, including their different designs and applications.
Related Components | Test Your Knowledge
sponsored by 
2. Objectives
Upon completion of this learning module, you will be able to:
- Understand the crystal structure of ferrite
- Describe the properties of ferrite
- Explain different types of ferrite
- Discuss the applications of ferrite
3. Basic Concepts
What is Ferrite?
Ferrites are nonconductive ferromagnetic ceramic compound materials having numerous combinations of iron oxides, such as Hematite (Fe2O3) or Magnetite (Fe3O4), and various other metals oxides, including manganese oxide (MnO), nickel oxide (NiO), zinc oxide (ZnO), and copper oxide (CuO). They are commonly used in electronic components due to their high electrical resistivity and low eddy current losses. The properties of ferrites vary depending on synthesis conditions, composition, sintering temperature, and grain size.
What’s the difference between a ferromagnetic material and a ferrimagnetic material?
Ferromagnetic materials exhibit a strong, permanent magnetic field. The magnetic moments of the atoms in a ferromagnetic material are aligned in the same direction, resulting in a net magnetic moment and magnetic ordering. Examples of ferromagnetic materials include iron, cobalt, and nickel.
Ferrimagnetic materials exhibit a weaker magnetic field compared to ferromagnetic materials. In ferrimagnetic materials, the magnetic moments of the atoms are aligned in opposite directions, but only partially canceled out. Their net magnetic moment and magnetic ordering are weaker than those of ferromagnetic materials. Examples of ferrimagnetic materials include magnetite (Fe3O4) and spinel (MgAl2O4).
How Are Ferrites Classified?
Ferrites are classified based on their magnetic properties and crystal structure. Generally, ferrites are divided into two types: soft ferrites and hard ferrites. Soft ferrites, such as zinc, cobalt, nickel, manganese, and magnesium, have low coercivity, meaning that their magnetism can change. Soft ferrites can act as conductors of magnetic fields. Hard ferrites, on the other hand, have high coercivity; they are used to make permanent magnets.
Ferrites can also be classified based on their crystal structure. Four groups exists: spinel, garnet, Ortho, and hexagonal, each distinguished based on the molar ratio of Fe2O3 to other oxide components present in the ceramic.
What is the Crystal Structure of Spinel Ferrites?
Spinel ferrite crystallizes in a cubic structure and has a closely packed arrangement of oxygen anions, in which 32 oxygen ions form each unit cell. The anions are packed in a face-centered cubic (FCC) array, leaving two types of spaces between anions: tetrahedrally-coordinated sites (A), surrounded by the four nearest oxygen atoms, and octahedrally-coordinated sites (B), surrounded by the six nearest-neighbor oxygen atoms. Figure 1 illustrates the positions of the four oxygen ions in each octant. The positions are the same in octants sharing only one edge and different in octants sharing a face. The figure further depicts the ionic positions at two adjacent octants, where the octant on the top contains the octahedral sites, and the octant on the bottom contains the tetrahedral sites. All ions are positioned along the diagonals of the octants, and the octant at the bottom contains a tetrahedral site at the octant center.

Figure 1: Crystal structure of Spinel ferrite. Image Source: Yu, Lichao, Applied Physics, 2020
What are the Magnetic Properties of Ferrites?
Ferrites exhibit a permanent type of magnetism referred to as ferrimagnetism, the phenomenon where the magnetic moments of atoms are aligned in both parallel and antiparallel directions. The overall magnetic field of ferrite is weaker than ferromagnetic materials because the magnetic field is partially canceled. Below the Curie temperature, ferrimagnetic materials exhibit a magnetic moment even without a magnetic field, similar to ferromagnetic materials and the hysteresis phenomenon. The paramagnetic behavior of ferrimagnetic materials above the Curie temperature is quite similar to ferromagnetic materials. The significant properties of ferrites include high electrical resistivity, low eddy currents and dielectric losses, high saturation magnetization, high permeability, moderate permittivity, and spontaneous magnetization. The saturation magnetization of ferrites is high in comparison with diamagnetic or paramagnetic materials, but as a ferrimagnetic, they exhibit saturation magnetization significantly lower than ferromagnetic material, such as amorphous or nanocrystalline alloys. In comparison with other materials considered magnetic materials, the saturation magnetization and permeability of ferrites is low.
How are Ferrite Magnets Made?
Ferrite manufacturing involves the following processes:
- Pre-Treatment: The pre-treatment process involves the conversion of metal hydroxides and metal oxides into oxides by sintering at 800-1000℃ after they are mixed and pelletized. The result is a calcined product which is subsequently mixed with water and ground to make a slurry.
- Spray Drying: The spray drying process converts the slurry into spherical powder using a spray dryer. The slurry is dispersed, and the surface tension produces spherical droplets dried with hot air.
- Sintering: The spherical powder is formed into a ferrite via sintering, the process of forming a solid object through pressure or heat. For ferrite, sintering requires a high temperature of 1000-1300℃ in an electric furnace with restricted oxygen concentration. This is the stage in manufacturing where ferrite achieves its final magnetic and mechanical properties.
- Deagglomeration & Classifying: At this point, the product produced in the sintering process undergoes deagglomeration, where particles that are clustered or clumped together are separated.
4. Analysis
In this section, we will discuss different ferrite designs, the applications of ferrites, and how to select the right ferrite for a particular application.
What are the Different Types of Ferrites?

Figure 2: Impedance curve of a WE-CBF HF ferrite bead.
The resistivity of a ferrite bead is strongest in a thin frequency band; its behavior can be described as frequency-dependent resistance. Above this frequency band, the impedance begins to appear capacitive.
Different types of ferrite beads are available:
- Flat Ferrites: Flat ferrites have a unique flat design, typically disk-shaped or rectangular (as opposed to cylindrical). They are used in applications where a flat shape is important, such as in planar transformers, power inductors, and filters. Flat ferrites are well-suited for electromagnetic emissions suppression and radio frequency interference.
- Ferrite Sleeves and Ferrite Rings: Ferrite sleeves or rings are cylindrical-shaped components made of ferrite material. The ferrite sleeve is placed around a cable or wire, acting as a filter that blocks high-frequency noise and allows only low-frequency signals to pass through. In order to maximize the benefits of interference suppression, the inner diameter should closely match the cable diameter. Ferrite sleeves and rings are used in a variety of applications including data communication systems, power supplies, and consumer electronics to improve signal integrity and reduce the impact of interference on the performance of the circuit.
- 6-Hole Ferrite Beads: A 6 hole ferrite bead consists of a cylindrical ferrite core with 6 through-holes running parallel to the axis of the cylinder. The bead is typically placed around a wire or trace in a circuit and acts as a low-pass filter, blocking high-frequency signals and allowing only low-frequency signals to pass through. The 6 holes in the bead facilitate the connection of the wire or trace to a circuit board. Ferrite beads are used in a variety of applications, including power supplies, data communication systems, and consumer electronics, in order to minimize the impact of electromagnetic interference (EMI) and radio frequency interference (RFI).
- Multiline Ferrites: A multiline ferrite refers to a type of ferrite material that has multiple lines of magnetic anisotropy, as opposed to single-line ferrites, which have just one line. This characteristic makes multiline ferrites suitable for a wide range of applications, such as broadband transformers and common mode choke applications, where high performance and efficiency are required. The impedance or current can be adjusted depending on the connection. Conversely, the appropriate connection can generate a double current-compensated broadband choke.
- SMD Ferrites: Surface mount ferrites or 'chip bead' ferrites consist of a multilayer flat metal coil (aka 'meander') embedded in Nickel-Zinc (NiZn) ferrite material. In this sense, an SMD ferrite is essentially an inductor with high resistive losses at frequencies approaching or higher than 100MHz.The two ends of the meander are connected to terminals that allow connection to PCB soldering pads. Since SMD ferrite uses flat wire windings, they have an extremely low DC resistance (few mΩ to 1Ω). Their use helps to avoid the problems of voltage drop and potential difference, particularly in DC applications.

Figure 3: SMD ferrite. Electromagnetic Compatibility - Without Equations. Chetan Kathalay, 2019
What is the Difference Between a Ferrite Bead and an Inductor?
Although a ferrite bead can be designed as an inductor, ferrite bead inductors do not behave as typical inductors. An inductor is often used to block alternating current (AC), so that direct current (DC) can pass through the circuit. Even though a ferrite bead is classified as an inductor, their frequency-impedance gives them different characteristics. The key differences between the inductors and ferrite beads are:
- A ferrite bead is an energy conversion (consumption) device, while an inductor is an energy storage component.
- Ferrite beads are mostly used in signal circuits for Electromagnetic Compatibility (EMC) countermeasures, while inductors are typically used in power supply filter circuits.
- Ferrite beads are typically used to suppress electromagnetic interference (EMI), while inductors are used to suppress conducted interference. Both can handle EMC and EMI issues.
Figure 4: Ferrite Bead vs. Inductor Impedance. Image Source: ROHM Semiconductor
- Ferrite beads are components with a low Q factor. Ferrite beads absorb more noise than inductors, and are thus more effective than inductors for reducing noise emissions over a broader range of frequencies.
Figure 5: Ferrite Bead vs. Inductor Impedance in the presence of DC. Image Source: ROHM Semiconductor
- When a DC current is passed, ferrite beads attain saturation, causing a decrease in inductance and shifting the resonance point to higher frequencies. In general, inductors are able to tolerate DC currents without a significant change in inductance.
What are the Applications of Ferrites in Electrical Engineering?
Ferrite beads are passive devices that filter out high-frequency switching noise/signals. Ferrite beads are used in a wide variety of applications.
- Common Mode Choke: Common mode chokes with a wide attenuation bandwidth are required to reduce common mode noise on data lines that are transmitting high-frequency signals. The presence of common mode noise can affect the performance of other electronic devices connected to the same power source, causing potential failures. Figure 6 illustrates the use of a ferrite as a common mode choke. I1 and I2 are differential mode currents, and Ic is a common mode current following a different return path. The abnormal current return path is facilitated by the parasitic capacitance Cc. Using ferrite beads with their high inductance will reduce the magnitude of Ic, particularly at high frequencies. Because the ferrite bead’s effectiveness in reducing common-mode current is dependent on frequency, the correct bead material must be carefully chosen in order to target the right frequencies.
Figure 6: Ferrite used in a common mode choke application. Image Source: Perez, Reinaldo. Wireless Communications Design Handbook: Interference Into Circuits: Aspects of Noise, Interference, and Environmental Concerns. Academic Press, 1998
- EMI Suppression: Ferrites are used for EMI suppression in electronic devices, where they effectively integrate high-frequency resistance into a cable to minimize common mode current and radiation. They act like a common mode choke and minimize the conducted and radiated emissions from the cable. Ferrite cores work as high-frequency resistors with no impedance at DC and lower frequencies. They significantly attenuate unwanted noise signals over 10MHz.
- Pulse Transformers: In some cases, the transistors that act as a switch to invert or form high-frequency square waves are free-running and do not require any timing mechanism. However, many transistors require a trigger; this signal can be generated by a pulse transformer. Pulse transformers operate at high frequencies and produce pulses of a constant amplitude. They typically contain small toroidal (or ring) ferrite cores.
- Switching Regulators: A switching power supply's output must have either highly controllable voltage limits or a good degree of regulation. To accomplish this, a device called a switching regulator is used. A switching regulator samples the output voltage, comparing it with a known voltage and detecting the difference. This difference is used to change the on-off time of the transistor and correct the voltage. Ferrite cores are often used in switching regulators.
- Input Filtering: Power supplies are designed for steady-state operation; however, transient conditions must also be considered. In practice, transient conditions such as startup, shutdown, and load transients are often far more stressful on the components of the power supply than operation in a steady state. To suppress high-frequency noise, chip bead ferrites are frequently placed at the input and output of power supplies.
Figure 7: Generic DC-DC converter with chip bead ferrite beads L1-L4 at the inputs and outputs
- Wireless Power Transfer: Ferrites are capable of confining and leading magnetic flux; thus, they can be used to improve performance in wireless power transfer (WPT) systems due to their high relative permeability and low loss tangent. Ferrite plates are placed at the bottom of the respective receiving coil and planar WPT receiver, in order to improve magnetic coupling and improve efficiency.
- Ringing Control: Ringing refers to undesired oscillation that can happen when a power semiconductor switch turns on or off in the presence of parasitic inductance and capacitance. Ferrites remove ringing in some applications, such as in power electronics or digital circuits.
How Do You Select a Ferrite Bead for a Circuit Design?
The selection of a suitable ferrite for an application depends on several factors:
- Materials: The material chosen is dependent on the frequency that requires the most attenuation. An ideal ferrite should offer the highest impedance levels at the interference frequencies, which usually cover a broad spectrum. Common ferrite materials include manganese zinc (MnZn) and nickel-zinc (NiZn) composites. MnZn is typically effective in the 0.1-MHz to the 1.5-MHz range (some can be effective up to around 10MHz). Performance in the highest-frequencies can be found with NiZn ferrite materials, which are effective at about 1 MHz up to 2 GHz due to their higher resistivity.
- Shape of the Core: The core is shaped to optimize suppression performance, as well as present a usable form factor for different applications. The cylindrical core is the most common ferrite geometry used in noise-suppression applications. Installing a ferrite core around a cable can effectively reduce both conducted and radiated electromagnetic emissions. The greater the length of the cylinder, the higher the impedance.
- Bias Current: The frequency response of ferrite can be altered when used in a circuit that surpasses its specified DC rating. As the DC bias increases, the magnetic material approaches saturation and its permeability decreases, causing part (or all) of the signal to fall into the non-linear region of the ferrite. This causes a considerable drop in the impedance within the ferrite’s rated bandwidth. This loss of impedance can be compensated for with an increase in the length of the core (the longer the core, the higher the impedance). An alternative way to reduce the negative effect is to introduce a small gap in the ferrite core (feasible in bisected types).
- Temperature: Temperature can play a significant role in ferrite selection. Ferrites have different temperature coefficients of permeability, which means that the magnetic properties of a ferrite material can change with temperature and affect performance. In general, ferrites with a lower temperature coefficient of permeability are more stable over a wide temperature range and are, therefore, more suitable for high-temperature applications. Additionally, some ferrites have a Curie temperature at which they lose their magnetic properties. Above this temperature, the ferrite is not useful.
- Relationship Between Impedance and Attenuation: Measuring ferrite impedance is one of the most common methods for selecting a ferrite. The impedance of a ferrite core is typically the most important specification for EMI applications, but in many cases, the amount of attenuation is just as relevant. The relationship between impedance and attenuation is expressed as follows:
Where A is attenuation in dB, and ZA, ZF, and ZB are source impedance, suppressor core impedance, and load impedance, respectively. Figure 8 illustrates a method for determining the attenuation of a ferrite core. Increasing the impedance of the cable ferrite will increase the attenuation for a given source and load impedance.

Figure 8: Determining the attenuation parameter of a ferrite core
How a Ferrite Bead is Used in a DC-DC Boost Converter
Figure 9 depicts the schematic diagram of an adjustable DC-DC boost converter that can be used for a variety of different voltage boost requirements. The circuit uses an LM2577 IC, a simple switcher and step-up boost regulator IC. The input voltage of the circuit is 3.5V to 40V DC. The regulated output voltage is set by resistors R1 and R2. A switch inside the IC closes between pins 4 and 3, causing current to flow through the inductor to the ground. After a few microseconds, the switch is released, and the inductor produces a back-EMF ‘kick.’ This result is a positive pulse with respect to the input voltage. This pulse charges the output capacitor via the Schottky diode-D1, which tends towards an equilibrium voltage.
The output voltage is monitored via the voltage divider R1/R2, causing the duty cycle of the switch oscillator to be continuously regulated in order to maintain a constant output voltage under varying loads. The maximum output voltage and current of the circuit are 60V and 5A, respectively. The ferrite bead reduces any switching noise that might be generated.

Figure 9: DC - DC Boost Converter Circuit Diagram. Designed using: pro.easyeda.com
5. Glossary
- Absolute permeability (μ or μabs): The ratio of magnetic flux density (B) to magnetic field strength (H) in a core medium. μ is a measure of the ability of a material to conduct a magnetic field.
- Anisotropy: The property of a material or substance having different physical properties along different axes. In the context of ferrites, anisotropy refers to the difference in magnetic properties along different crystal axes.
- Attenuation: The decrease in signal magnitude in transmission from one point to another. It is a scalar ratio of the input magnitude to the output magnitude, expressed in decibels (dB).
- Curie Temperature (TC): The temperature at which certain magnetic materials undergo a phase transition from a feromagnetic or ferrimagnetic state to a paramagnetic state. Also called the Curie Point.
- Diamagnetism: A diamagnetic substance is one whose atoms have no permanent magnetic dipole moment. When an external magnetic field is applied to a diamagnetic substance, such as bismuth or silver, a weak magnetic dipole moment is induced in the direction opposite the applied field.
- Eddy Currents: Conductor currents that circulate like eddies swirling in a stream. Eddy currents are induced by changing magnetic fields and flow in closed loops, perpendicular to the magnetic field. They can be created when a conductor moves through a magnetic field, or when a magnetic field that surrounds a stationary conductor varies.
- Electromagnetic Compatibility (EMC): The ability of electrical and electronic equipment to function reliably in their electromagnetic environment, without being affected by or causing interference to other electronic devices.
- Inductance (L): The ability of a component or circuit to store energy in a magnetic field when electric current flows through it, opposing changes in current flow. The unit of inductance is the henry (H).
- Magnetic constant (μ0):A scalar constant that gives the permeability of vacuum or free space (1.256 6370614 μH/m)
- Magnetic field: A constituent of an electromagnetic field and is characterized by the magnetic field strength (H) together with the magnetic flux density (B). Both H and B are vector quantities.
- Magnetic flux (ɸ): A measure of the amount of magnetic field passing through a given surface. It is defined as the product of the magnetic field strength and the area of the surface perpendicular to the field. The unit of magnetic flux is the weber (Wb).
- Q Factor (quality factor): A dimensionless parameter that describes the performance of a coil or resonant system. The Q factor is a measure of the energy stored in a resonant system relative to the energy lost per cycle of oscillation.
- Relative permeability (μr): The ratio of the absolute permeability μ of the core medium to the magnetic constant μ0.
- Schottky diode: A metal-semiconductor junction diode with less forward voltage drop than the P-N junction diode and a fast switching speed.
*Trademark. Würth Elektronik is a trademark of Würth Elektronik Group. Other logos, product and/or company names may be trademarks of their respective owners.

For more ferrite products Shop Now
Test Your Knowledge
Passives I
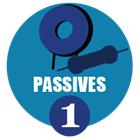
Complete our Essentials: Passives I course, take the quiz, and leave your feedback to earn this badge.
Are you ready to demonstrate your ferrites essentials knowledge? Then take a quick 10-question multiple choice quiz to see how much you've learned from this module.
To earn the Passives 1 Badge, read through the learning module, attain 100% in the quiz at the bottom, and leave us some feedback in the comments section below.
Top Comments